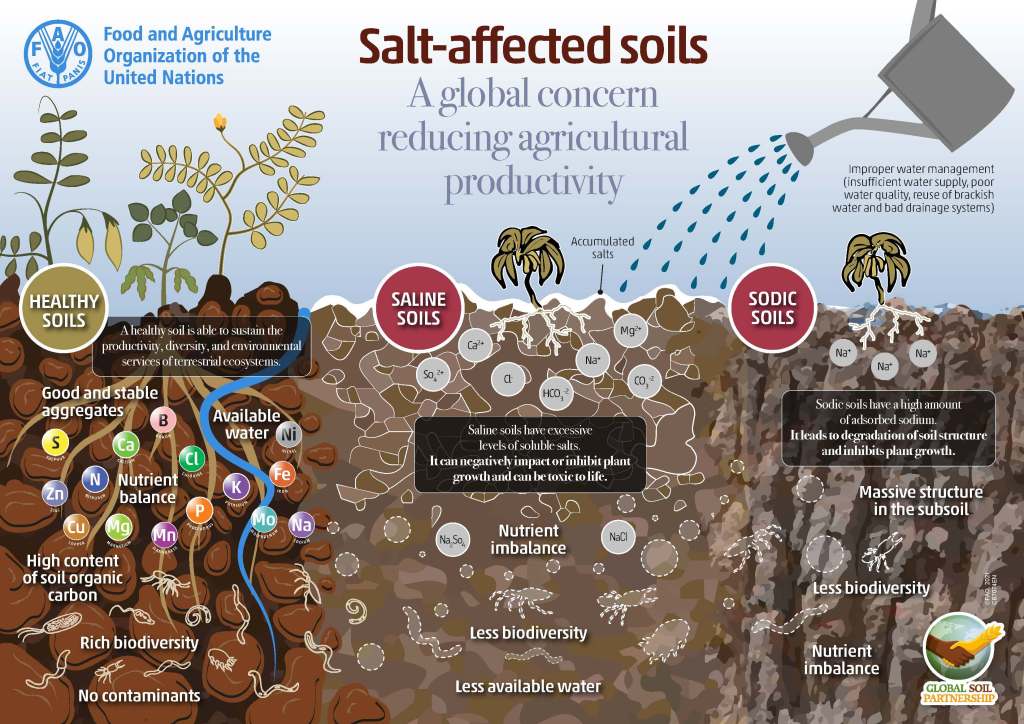
Introduction
Every December 5 the Food and Agriculture Organization (FAO) of the United Nations recognizes World Soil Day. Through this celebration, the FAO acknowledges the importance of soil as a resource – in other words critical infrastructure – and sets a theme of soil science education for the subsequent year. This year the said theme is “Halt Soil Salinization, Boost Soil Productivity.”
First, salinization is the process by which soils become more concentrated in dissolved salts (saline soils) or sodium chloride specifically (sodic soils). Both salinity and sodicity decrease soil quality, increase soil susceptibility to erosion, and decrease plant productivity. See https://youtu.be/kQcax3Rv4oA for the FAO’s quick primer on salinization.
Given the topic of Lynn’s post on soil as infrastructure last month, the recognition of World Soil Day earlier this month, and the threat that anthropogenic salinization poses to soil’s critical function of supporting the earth’s ever-growing population, I think that the FAO’s World Soil Day theme is an apt topic to further expand upon Lynn’s argument.
A key piece to this blog post about the importance of curtailing salinization, and the recognition of soils as critical infrastructure, is the acknowledgement that soils are and will continue to be the media through which the world’s population feeds itself. Any threat to soil is a threat to humanity – especially those in less industrialized and poorer regions of the world.
Salinity and Sodicity in Soils
Saline soils, often identified by white salt crusts at the soil surface (Figure 2 ), are problematic because the dissolved salts inhibit plant uptake of water. Plant roots utilize osmotic potential – the differential of dissolved salts between the plant’s cells and the soil – for water uptake. In saline soils the osmotic potential favors water flowing out of the plant and back into the soil; the opposite of what most plants need for water uptake. Without special evolutionary tricks to take up this salty water, plants languish and eventually die.

By USDA NRCS Montana – Saline Seep02.tif, Public Domain, https://commons.wikimedia.org/w/index.php?curid=68798631
In sodic soils, the sodium chloride acts to destabilize soil aggregates. Soil aggregates are key to soil drainage, soil water holding capacity, plant root penetration, and organic matter stabilization (an important sink for atmospheric CO2).
When rain falls on a sodic soil, the surface aggregates disintegrate into constituent soil particles. These soil particles are either carried by water to become sediment in streams and lakes, or further percolate into the soil profile. In the soil profile, the soil particles ‘plug-up’ pores to form a hardpan (a block of soil with no structure). This hardpan results in poorly drained soils that cannot support plant life.
Sodic soils create a positive feedback loop of soil erosion. Sodium chloride causes aggregate instability during rain events –> soil particles erode from the soil surface and plug soil pores resulting in a hardpan –> during next rainfall event water cannot infiltrate the harpan, which results in overland water flow –> this overland flow drives more soil erosion.

Anthropogenic Soil Salinization
Saline and sodic soils exist naturally in small pockets across the globe. For example, naturally sodic soils are scattered across Oklahoma (Figure 4). Additionally in Oklahoma, the Salt Plains Wildlife Refuge is an inland saline ecosystem. Inland sodic and saline soils, like those in Oklahoma, generally form from salt-rich marine deposits in arid and semi-arid regions.

However, human activities also increase the prevalence of such salt-affected soils (Figure 5). Land use change, intensification of agriculture, improper irrigation practices, improper disposal of saline industrial wastewater, and anthropogenic saltwater intrusion (e.g., groundwater pumping in coastal areas, sea level rise resulting from climate change), all have the capacity to induce soil salinization (Gorostiza & Sauri, 2019; Litalien & Zeeb, 2020).
As industrialized agriculture expands to semi-arid and arid climates in order to meet the nutritional needs of the global population, salinization will increase due to the removal of native plant species and the use of irrigation to support crops.
Native plants in dry soils are deeply rooted to access groundwater. However, when native plants are cleared and replaced with shallowly rooted cash crops, the groundwater level rises. Without the deep roots using the groundwater, the amount of water in the subsurface increases; the greater volume of below ground water drives the water table shallower. As groundwater rises, soluble salts common in arid and semi-arid soils dissolve, which increases groundwater salinity.
This newly saline water is pumped and delivered to crops as irrigation. When irrigation is over-applied, percolating waters dissolve more soluble salts, and reach the (now shallow) groundwater to further increase subsurface salinity (Jobbágy et al., 2021). This process drives salinization across Central and Western North America, the Pampas of South America, much of India, parts of Southern Africa, and the majority of Eastern Asia.
Such agriculturally induced salinization is not a new problem – there is a consensus that from 3500 to 1250 BCE overuse of irrigation in the floodplains of the Tigris and Euphrates resulted in salinization, loss of agricultural productivity, shift of production to salt-tolerant barley, and eventual abandonment of agricultural production altogether (Jacobsen & Adams, 1958).
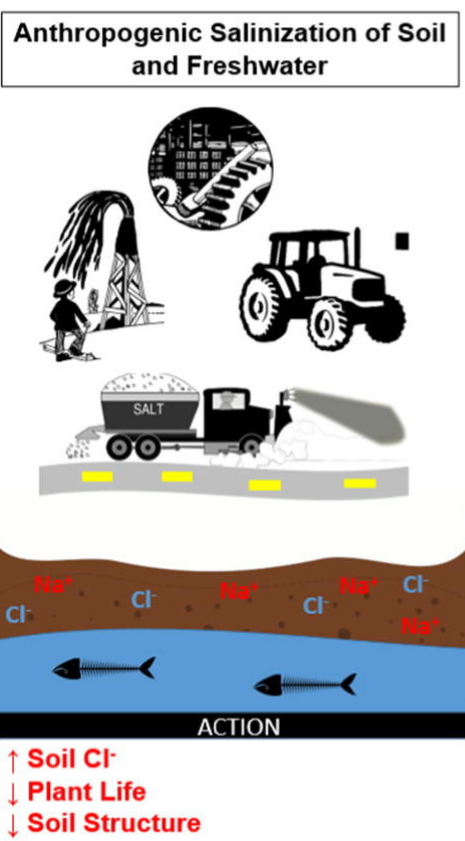
Another example of anthropogenic salinization, particularly relevant to Oklahoma, is the land application of produced water and mud from conventional and unconventional oil and gas operations. These operations are estimated to produce about 600 – 800 billion gallons of wastewater a year in the United States alone (Kondash et al., 2017). While not uniform in chemistry, all produced water is highly saline.
In Oklahoma, much of the produced water is disposed through application on agricultural and range lands. Even though produced water is applied as a dilute solution, there is evidence that land application increases symptoms of soil sodicity, decreases soil health and microbial diversity, and can over time decrease crop yields due to salinity (Whitaker et al., 2017; Miller et al., 2020).
What Can be Done?
Unfortunately, there is no easy fix for soil salinization, as its root is that we must stretch the earth’s finite natural resources to support increasingly more people. However, the deleterious effects of salinization can be reduced through careful management of water resources and ecological restoration.
Effects of sodicity can be ameliorated by increasing soil organic matter content through use of cover crops and applying amendments that stabilize soil aggregates (e.g., gypsum promotes soil ‘flocculation’). The cover crops, soil organic matter, and flocculating agents decrease the potential for aggregate breakdown during rainfall events and therefore limit soil erosion and hardpan formation.
Salinity in soils can be managed through proper management of water table level. The first step is to reestablish the native, deeply rooted plants that will maintain groundwater lower than the salts in soil profiles. After this, conservation irrigation should be employed such that irrigated waters sufficiently supply water to the rooting zone but never reach the groundwater. In these soils, production of (and development of new) salt tolerant varieties of crops is recommended.
While these solutions are viable for large scale, industrialized agriculture, the small-scale farms that feed much of the world’s population have struggled to adapt. These producers do not have access to the technology, research-based agricultural education, and new salt-tolerant crop varieties that can make saline soils productive.
If we want to sustainably and equitably meet the demand for greater agricultural production – while also maintaining the integrity of soil as critical infrastructure – we must develop and disseminate new methods of water and land management to curtail soil salinization.
Acknowledgement
Thanks to Dr. William Miller – my early mentor and Soil Erosion and Conservation Professor at the University of Georgia – for his pioneering work on soil dispersion in humid environments. I was reminded of many of his fieldtrips and lectures while writing this blog.
References
Gorostiza, S., & Saurí, D. (2019). Naturalizing pollution: a critical social science view on the link between potash mining and salinization in the Llobregat river basin, northeast Spain. Philosophical Transactions of the Royal Society B, 374(1764), 20180006.
Jacobsen, T., & Adams, R. M. (1958). Salt and silt in ancient Mesopotamian agriculture. Science, 128(3334), 1251-1258.
Jobbágy, E. G., Giménez, R., Marchesini, V., Diaz, Y., Jayawickreme, D. H., & Nosetto, M. D. (2021). Salt Accumulation and Redistribution in the Dry Plains of Southern South America: Lessons from Land Use Changes. In Saline and Alkaline Soils in Latin America (pp. 51-70). Springer, Cham.
Kondash, A. J., Albright, E., & Vengosh, A. (2017). Quantity of flowback and produced waters from unconventional oil and gas exploration. Science of the Total Environment, 574, 314-321. https://doi.org/10.1016/j.scitotenv.2016.09.069
Litalien, A. & Zeeb, B. (2019). Curing the earth: A review of anthropogenic soil salinization and plant-based strategies for sustainable mitigation. Science of The Total Environment, 698, 134235. https://doi.org/10.1016/j.scitotenv.2019.134235
Miller, H., Dias, K., Hare, H., Borton, M. A., Blotevogel, J., Danforth, C., Wrighton, K. C., Ippolito, J. A. & Borch, T. (2020). Reusing oil and gas produced water for agricultural irrigation: Effects on soil health and the soil microbiome. Science of The Total Environment, 722, 137888. https://doi.org/10.1016/j.scitotenv.2020.137888
Whitaker, A., Penn, C. & Warren, J. (2016). Surface application of a saline-sodic oil & gas drilling waste to winter wheat (Triticum aestivum L.). Geoderma, 274, 97–103. https://doi.org/10.1016/j.geoderma.2016.03.024
Caitlin Hodges (ORCID: (https://orcid.org/0000-0003-3960-5771) is an Assistant Professor of Critical Zone Geoscience in the School of Geosciences at the University of Oklahoma.